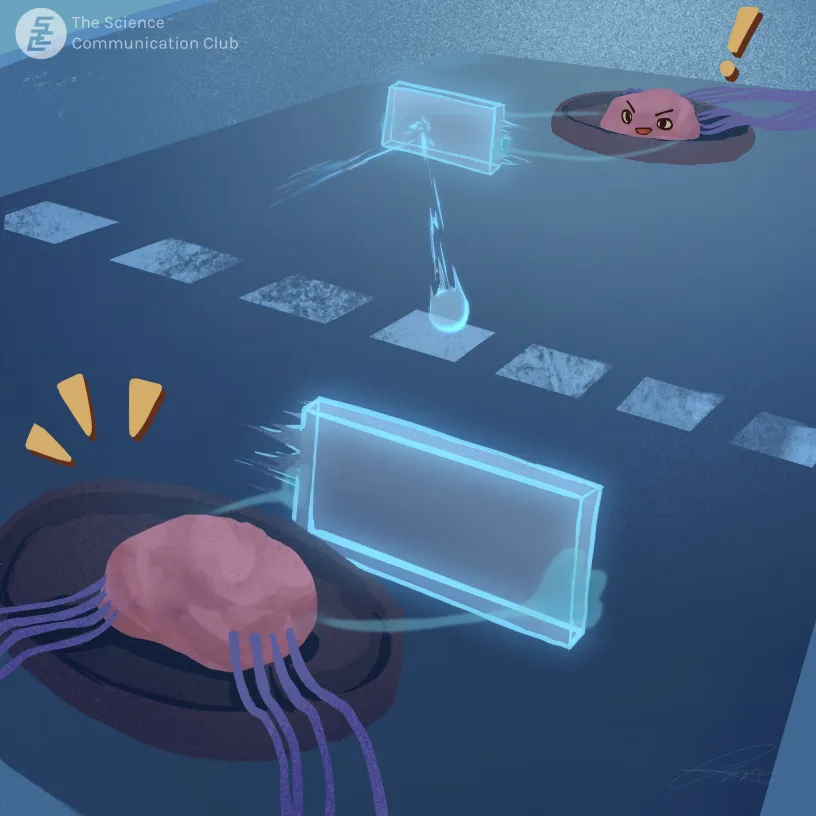
Written by Parmin Sedigh
Illustrated by Crystal Yang
How good are you at the arcade game Pong? I don’t think I’ve ever played it, but playing the game has become a rite of passage for machines or systems trying to display their “intelligence.” Google-owned DeepMind mastered it in 20151, and training artificial intelligence (AI) models to play it is even a bonus assignment in a computer science course at the University of Toronto2. We’ve all become used to AI performing human-like tasks. But can you imagine a group of cells in a dish also being capable of playing the game?
In 2022, Brett J. Kagan and his colleagues at Cortical Labs in Australia published a paper describing a system they’d created called DishBrain3,4. We often think of neurons firing as synonymous with complex thinking. But did you know that neurons grown in a dish fire too—and that this can be used to help train them?
After connecting the neurons to a computer, scientists looked at how they fired. If the neurons’ pattern of firing resulted in a desirable outcome in Pong (i.e., the ball hit the paddle), the researchers would deliver an input that told the neurons “Good job, keep it up!”3. If the ball didn’t hit the paddle, they would do the opposite3,4.
Amazingly, they found the neurons’ performance in playing Pong improved when doing this. The neurons were learning. This fascinating paper naturally piqued the interest of other scientists and soon, researchers were coming together in laying the groundwork for a brand-new field: Organoid intelligence (OI)5.
To understand OI, we first need to break down the term. Organoid refers to 3D models of cells. Traditionally, cells are grown (or cultured) in Petri dishes in a single layer, meaning the system as a whole is 2D (though the individual cells are, of course, 3D). But, as you and I both know, the organs in our bodies are not 2D. Imagine trying to explain a sphere to someone who can only see in 2D; it would be really difficult if not downright impossible. Putting it more scientifically, 2D cell cultures aren’t nearly as good at capturing real-body processes as organoids6.
Interestingly, organoids are nothing new. The first organoids were created and described in 20097. What makes the OI field unique is the intelligence aspect. The field combines sensors and AI to create biological computers. Instead of silicon chips and a motherboard, we may one day be using organoids as the motherboard for our computers.
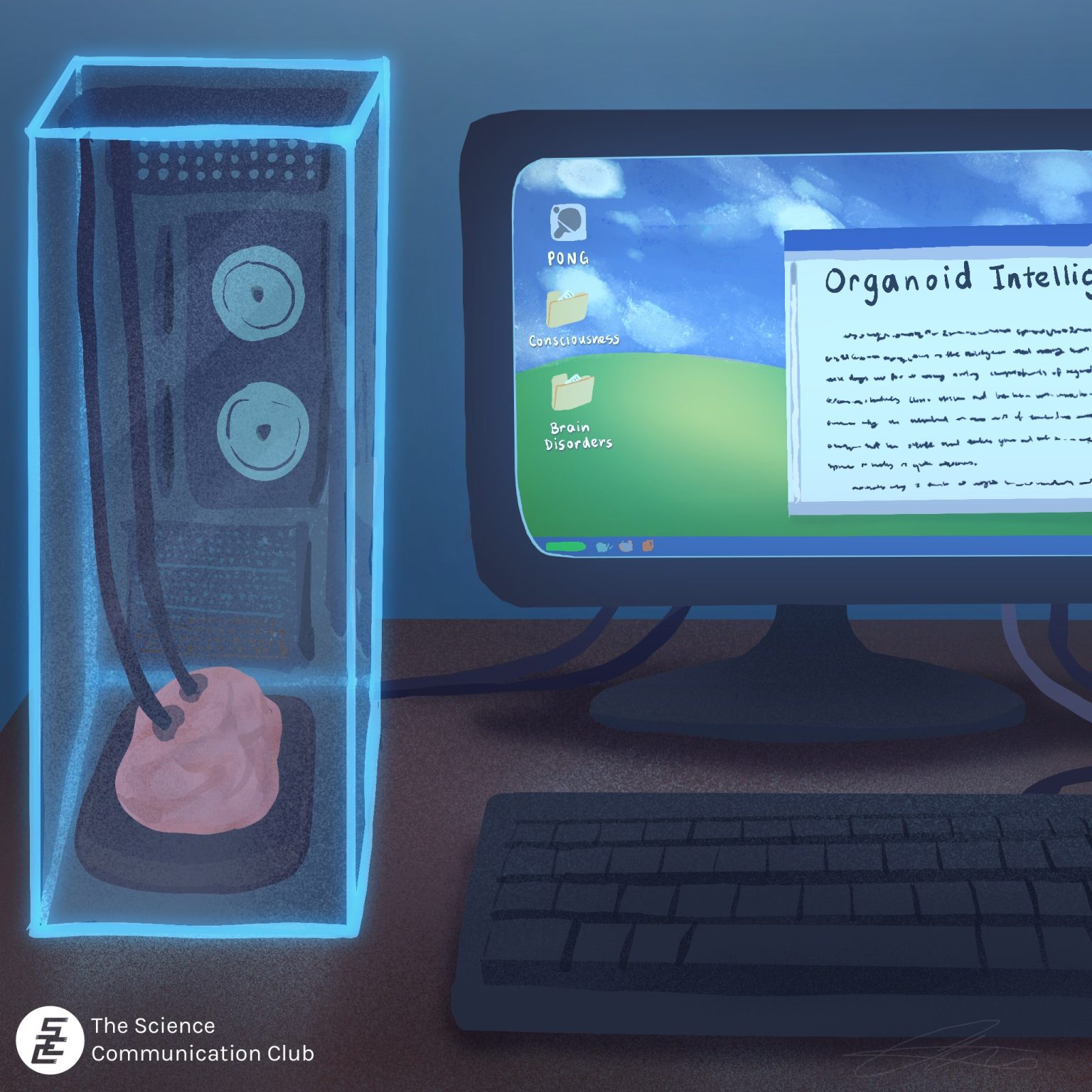
It’s important to note that the OI field is very new, and there’s still a lot to learn—not only for us curious minds but also for researchers whose full-time job is working at the intersection of biology and computing. In fact, you could truthfully claim to have read all of the literature within the field; the number of papers with the term “organoid intelligence” in the title can be counted on two hands. Such a claim would be absurd in most other fields. So, if you don’t have plans this weekend, set aside a few hours and read all of the papers in the field. Then feel free to tell everyone you know that you’re an expert in OI before the field expands!
Before we get carried away, let’s take a step back. Why is OI even necessary? Despite the revolutionary power of our computers, they are limited in ways our brains simply aren’t. For instance, humans can learn to tell the difference between a dog and a cat using just a few samples, but computers need significantly more5. As well, our brains need much less energy to do the same amount of work as a traditional computational system5. And our brain is able to process multiple things at the same time while classical computers—the ones you and I have—can only perform tasks sequentially5. Due to all of these advantages of OI, OI could one day replace the computers we use today.
Apart from computation, OI systems can be used to study learning, consciousness, and disorders relating to the brain like neurodegenerative disorders (e.g., Parkinson’s disease and Alzheimer’s disease) and neurodevelopmental disorders (e.g., autism spectrum disorder)5.
By now, we’ve all likely had the same thought: This seems interesting but what about the ethical implications? To be clear, it is not scientists’ goal to create conscious organoids. But it’s still possible this will occur without their knowing. During a panel discussion with the authors of the first official OI article as well as Karen Rommelfanger, an ethicist, Rommelfanger posed an important question: Would we actually know if organoids felt pain8? In other words, do we have the tools to measure this? These are questions we must answer now both because it is the morally sound course of action and because not doing so would erode the public’s trust in this fast-growing field. It’s incredibly encouraging that scientists are having these critical conversations early on in the development of the field.
The field of OI is indubitably full of excitement and potential. It promises to fundamentally transform our understanding of computation. Despite this, I must agree with Lena Smirnova and her colleagues when they write that “we are entering terra incognita.”
Sources:
1. Gibney E. Game-playing software holds lessons for neuroscience. Nature. 2015;518(7540):465–466. doi:10.1038/518465a
2. AI Engines for Pong. [accessed 2023 Nov 10]. https://www.cs.toronto.edu/~guerzhoy/niftypong/
3. Kagan BJ, Kitchen AC, Tran NT, Habibollahi F, Khajehnejad M, Parker BJ, Bhat A, Rollo B, Razi A, Friston KJ. In vitro neurons learn and exhibit sentience when embodied in a simulated game-world. Neuron. 2022;110(23):3952-3969.e8. doi:10.1016/j.neuron.2022.09.001
4. Ledford H. Neurons in a dish learn to play Pong — what’s next? Nature. 2022;610(7932):433–433. doi:10.1038/d41586-022-03229-y
5. Smirnova L, Caffo BS, Gracias DH, Huang Q, Morales Pantoja IE, Tang B, Zack DJ, Berlinicke CA, Boyd JL, Harris TD, et al. Organoid intelligence (OI): the new frontier in biocomputing and intelligence-in-a-dish. Frontiers in Science. 2023 [accessed 2023 Nov 10];0. https://www.frontiersin.org/journals/science/articles/10.3389/fsci.2023.1017235/full. doi:10.3389/fsci.2023.1017235
6. Jensen C, Teng Y. Is It Time to Start Transitioning From 2D to 3D Cell Culture? Frontiers in Molecular Biosciences. 2020 [accessed 2023 Nov 10];7. https://www.frontiersin.org/articles/10.3389/fmolb.2020.00033
7. Sato T, Vries RG, Snippert HJ, van de Wetering M, Barker N, Stange DE, van Es JH, Abo A, Kujala P, Peters PJ, et al. Single Lgr5 stem cells build crypt-villus structures in vitro without a mesenchymal niche. Nature. 2009;459(7244):262–265. doi:10.1038/nature07935
8. Thomas Hartung and colleagues | The future of organoid intelligence | Frontiers Forum Deep Dive 2023. 2023. https://www.youtube.com/watch?v=3dIjWDCchtc